The next frontier includes 3D-printed materials and antennas
Figure 1: Turbomachinery components like those shown here are typically produced using laser metal fusion. (All images provided by TRUMPF Inc.)
What do I think about space exploration? If you ask me that question and get me started on that topic, I probably won’t be able to stop. Since the new space race has begun, we are starting to see “new space” companies pop up that are basically tech startups: highly motivated, enthusiastic, driven individuals who are committed to their mission and will do “what’s right” to achieve their goals. Additive manufacturing (AM), or 3D printing, is a tool they can use to help achieve their goals. For metallic components on spacecraft or even on the launch pad, AM is, simply put, propelling the next generation of space exploration.
In the last few years, we have seen tremendous success in the public and private space exploration industry. The legendary William Shatner (Captain Kirk of “Star Trek” fame) took a ride on Blue Origin’s New Shepard vehicle when he was 90, becoming the oldest person to go to space. SpaceX’s Starship has a new tentative launch date for late 2022. Virgin Orbit launched satellites from the Mohave Air and Space Port, and they are on target to launch from the U.K. in coming months. Firefly had its first successful flight, and Astra also returned to launching from its Alaska facility. NASA’s Artemis mission got underway and, though pushed back, will resume when it is safe to do so. Many others continued making progress, including Rocketlab, ABL Space, Stoke Space, Venus Aerospace, and Firehawk.
The conversation of using AM for space exploration has continued unabated. For example, Relativity Space announced its intention to launch in late October 2022. This is significant, given that a large proportion of its vehicle is produced using AM processes, such as wire-arc additive manufacturing (WAAM) and laser-powder-bed fusion—also called laser metal fusion (LMF).
Many other space flight companies—old and new—are using AM processes for structural components, fuel tanks, barrels, and propulsion devices. The classic rocket engine lends itself well to 3D printing, and laser metal fusion is appropriate to produce turbo machinery, injectors, combustion chambers, and nozzles.
On the other end of the space spectrum, the very payloads that are being delivered to space can also be produced via AM. A satellite antenna, for example, is a perfect metallic component to take advantage of 3D printing to make it better, faster, and more reliable. Throughout 2022, I’ve been increasingly impressed by the ingenuity and savvy of AM users in producing parts for this industry, and marveled at this in a recent podcast conversation with Paul Gradl, a frequent collaborator from NASA and fellow space geek.
In this feature, we’ll detail a few obvious examples of AM for space exploration—and why these developments are very exciting.
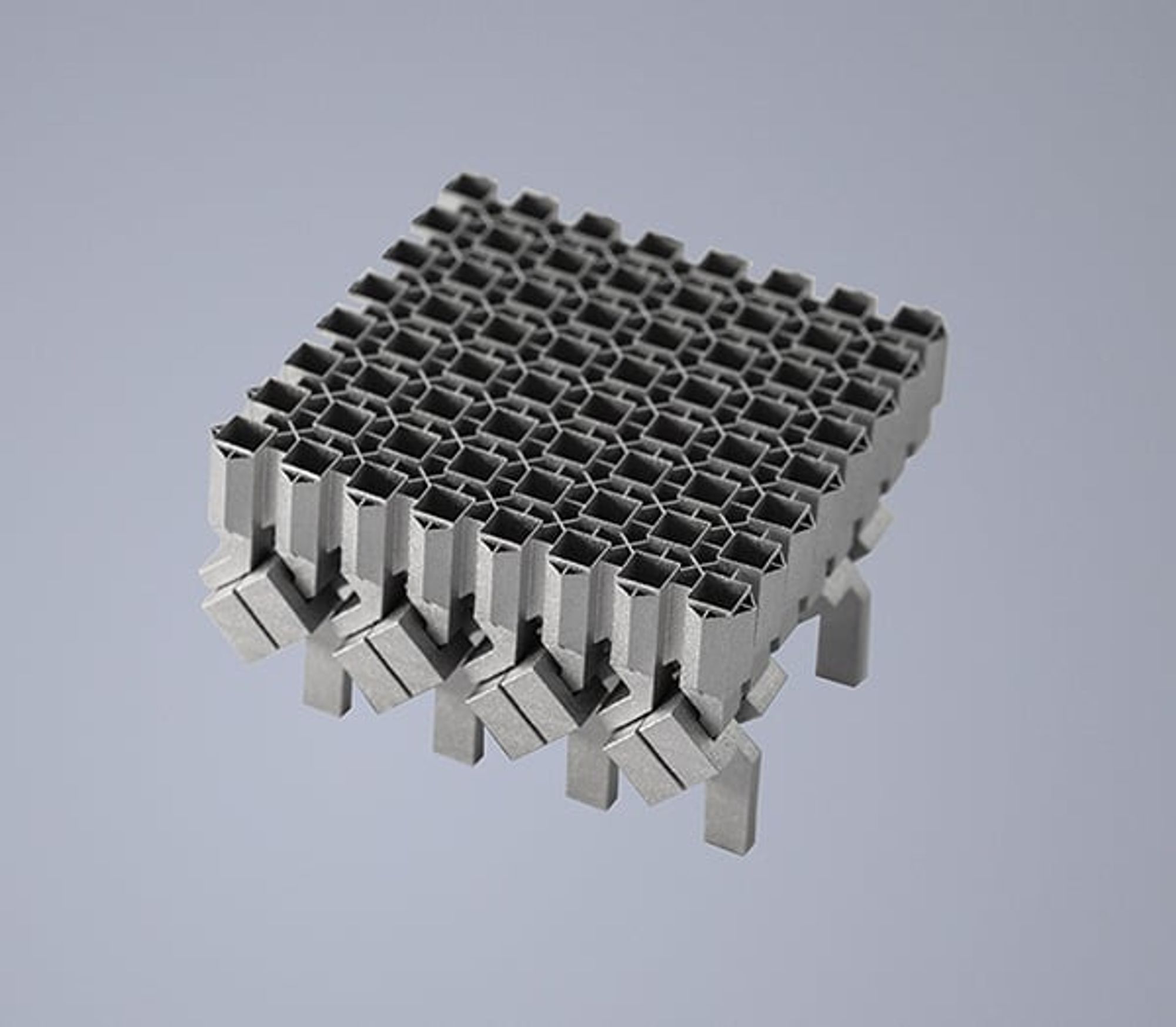
Figure 2: Laser metal fusion was used in the manufacturing of this satellite antenna.
Satellite Antenna
In an era in which we are all addicted to our phones, we’ve discovered that people simply cannot deal with not being connected. Apps on our phones, watches, goggles, and in our cars use global positioning satellites to provide internet access, music, video, and general connectivity. Rural communities that have been underserved by a lack of cell phone coverage in the past can now utilize satellite constellation networks for their connectivity. Students from lower-income families can now study and complete their homework online, allowing them to receive education on a more equitable basis. Whatever the need is, all these connectivity requirements mean that more satellites, particularly CubeSats and constellations, are needed. Fortunately, laser metal fusion is a great solution to speeding up the production and quality of these parts.
Figure 2 shows a graphic of a 3D-printed RF antenna for the Ka band. There are some excellent benefits of producing satellite antenna with LMF. The complex design can be easily translated into scanned movement of the laser beam to create shapes that would be difficult to manufacture with traditional processes. In this example, we see a part in AlSi10Mg, which is a well-understood aluminum alloy that prints well and reliably in a wide variety of laser powder bed fusion machines across the industry. The monolithic design can include features such as horns, filters, and waveguides, while actually reducing input loss.
If a small enough spot size is used, very fine wall thickness (down to 0.1 mm) can be obtained with excellent surface finish (6-7 mm Ra). Depending on the size of the part and the size of the machine, multi-laser machines even have a productivity benefit in that they can use the full size of the build plate to print multiple parts in a single cycle, without worrying about laser overlap and unexpected start-stops. If we continue developing LMF process reliability and quality, it stands to become the go-to process for manufacturing satellite components.
C103 Niobium Alloy for Engine Nozzles
Any material that is difficult to produce in traditional mill product form could be considered a candidate for AM processing as long as feedstock is available. A particular high-temperature legacy metal from the original space race, the niobium-based C103 alloy is a case in point. Second-stage vacuum engines for spacecraft and some afterburners on military aircraft have been developed using C103, as it has temperature resistance to a whopping 1,370° C.

Figure 3: Laser metal deposition is an ideal method of creating complex nozzle structures.
However, the traditional mill product, sheet metal, is difficult to produce in this material, as it goes from an electron beam primary melt, followed by vacuum-arc remelt “EB-VAR” ingot, then forged and rolled to sheet. This process would take upwards of 35 weeks from order entry to sheet metal appearing on the dock—an incredibly long lead time that does not include forming, water-jet cutting to shape, and EB or TIG welding to the final configuration, and then coating. Moreover, the thin gage sheet sections require an extreme handling process as they are incredibly sharp and can injure operators. Why not simply 3D print this material instead?
This is easier said than done. The highly arduous process of obtaining sheet metal C103 is only slightly better than producing powder or wire. However, AM processing using LMF of C103 powder has been successfully demonstrated. The benefits of creating parts with C103 powder are improved lead time and a more streamlined process. With 3D printing, only the needed powder is used, and there isn’t latent yield loss with cutting sheet drops. You can skip the forming and EB/TIG welding operations—you print your part, and voila. You also don’t risk injuries from handling sharp cut edges. For small propulsion devices such as satellite thrusters, laser metal fusion is an ideal process for the build volumes, again taking advantage of multi-laser machines to print multiples if they can all fit on the same build plate.
The other good news is that work has begun on wire additive processes, although there clearly needs to be greater feedstock availability for this to take off. I recently heard an argument from the other side of the materials science spectrum, asking why we continue to use C103 when there might be something better in a niobium or other refractory metal alloy that does the job of temperature resistance even better and cheaper. It’s obvious that the scope of improvement on this subject is wide open and fertile ground for materials development with ICME methods (thermodynamic models and software tools).
Green Lasers on the Bright Side of Light
AM processes involving lasers have all used infrared (IR) wavelengths to rapidly melt powder. But a technology from the world of cutting and welding, which utilizes laser wavelength in the visible spectrum of light, is also possible: green laser light. At 515 nm—opposed to IR’s 1030-1064 nm—green laser light was used for cutting and welding highly reflective sheet metal such as copper and its alloys.
While blue lasers have an even shorter wavelength, green laser technology currently is more developed and readily available. The highly reflective materials also extend past copper to some aluminum alloys, gold, silver, and platinum. With an infrared laser, these materials are difficult to couple with the beam. This tends to scatter the laser light, causing energy loss, which means more energy is required to pump into the material. What results is spatter, melt pool instability, and poor resultant cut or welded finishes.

Figure 4: This GR Cop engine component was produced via laser metal deposition with a green laser.
A green laser with a shorter wavelength has less reflected laser light, i.e., more of the laser energy can penetrate the metal, resulting in less spatter and a more stable melt pool. Translating this to 3D printing was an obvious choice. For heat exchanger components or anything requiring the higher thermal and electrical conductivity that copper allows, the resultant printed parts have higher density, less spatter, a higher as-printed density (lower porosity), and a better surface finish. Incredibly, the consumer jewelry industry has also benefited—most of the noble metals are expensive, so the ability to save on waste is advantageous for these materials. When extended to LMF machines, another aspect of using IR lasers, which could be detrimental for highly reflective materials, would be the amount of debris that collects on the inside of the machine build chamber, causing damage to lens covers and filters. In this case, using a green laser becomes even more attractive when productivity needs to be considered (i.e., less time required for machine clean-out, changing lens covers less frequently, and extending the life of the laser optic).
Recent developments in the rocket engine propulsion realm have included copper alloy engine component manufacturing by LMF, whether it be in C18150 (CuCrZr) or GR Cop 42 or 84. Copper alloy combustion chambers allow exceptional thermal conductivity but at the expense of some strength; therefore, a combination with a nickel-based superalloy to provide additional strength would be an excellent solution. With the limit of current LMF machines with infrared lasers topping out at 800-1000 mm maximum Z-height size, designers are pushing the very limit of what is possible. The bigger the engine, the greater the thrust output and the larger the payload or higher orbit that can be achieved.

Figure 5: In this example, laser metal deposition created this series of grid fins, a task that would have been far more challenging with conventional means.
Imagine a world where the free-form nature of laser metal deposition (LMD), also known as directed-energy deposition (DED), allows users to break free of the 800-1000-mm Z-height maximum. A 3D-printed rocket combustion chamber or even a full-size nozzle could be produced. Figure 3 shows (on a smaller scale) where tilting and moving a part free of a fixed build plate will allow overhangs to be dealt with, while, if a green wavelength laser would be used, more efficient printing process with better as-printed properties would result. Figure 4 shows a GR Cop 42 printed with a green laser in a laser metal fusion process. For a part about 15’’ [381 mm] tall, this print took 52 hours to print at 650 Watts, saving a great deal of laser energy and efficiency with an excellent surface finish. We can expect to see more parts produced with this process very soon, and hot firing any of these components will tell us more about the overall efficiency of the part and its ability to produce thrust.
Finally, we could also use the free-form AM processes like WAAM, laser-wire, laser hybrid-arc, or LMF to create large structures, perhaps for items on the launch pad that never leave the ground, or other flight articles. Figure 5 shows a laser metal fusion process used to create a grid fin, which is a structure on a missile or rocket that is used as an airbrake. It can move and rotate with pitch and yaw to deal with airflow, such as slowing down a recovered re-usable rocket’s first stage on re-entry. Traditionally these parts can be made by casting, so if saving lead time matters, then why not 3D print it?
As the Artemis program ramps up, it is only a matter of time before we see the first woman walk on the moon. Without a doubt, she and her colleagues will do so, with the help of additive manufacturing to get them there and back.