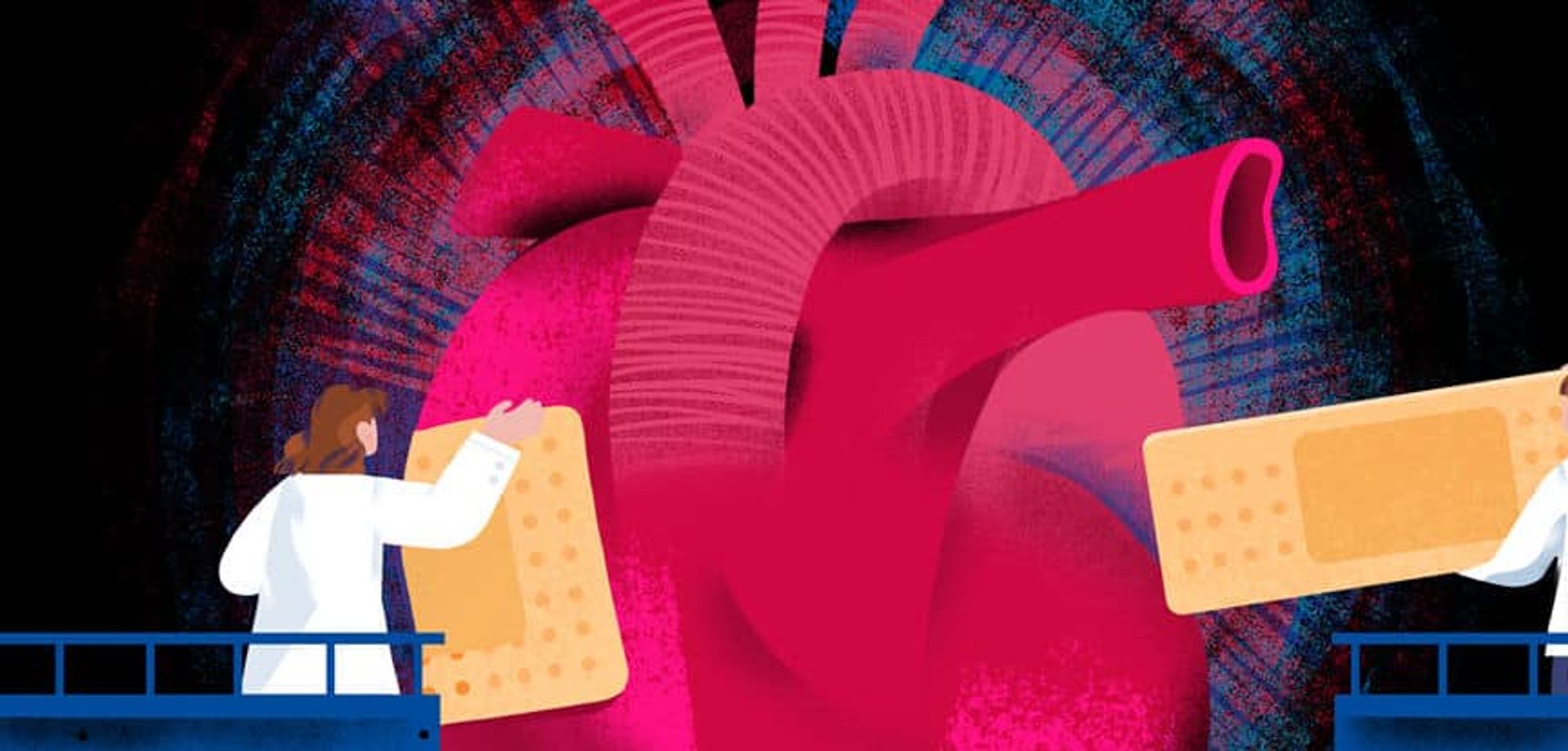
Scientists are increasingly realizing the potential of biomaterials to help with many tasks. A key area biomaterials are being used in is tissue engineering and regeneration, something several biotechs and academic labs are currently exploring.
Biomaterials are materials or substances designed to function in a biological environment, which may or may not be made of biological material. Many are inspired by natural phenomena such as how mussels stick to rocks or the glue-like secretions of the Californian sandcastle worm, whereas others focus more on how to solve biological problems using artificial polymeric biomaterials as templates or implants to assist with tissue engineering and regeneration.
Biomaterials are particularly well suited to being used in tissue engineering to restore, improve or replace damaged or defective tissues or organs in the body. Tissue engineering and regeneration have a lot of promise to solve difficult medical problems, but they form a complex field.
Therapeutic products can be expensive and time-consuming to develop and test. They are also relatively new to regulators, which can make gaining approval for therapeutic use difficult. Despite this, developments in molecular biology, such as next-generation sequencing, have helped drive tissue engineering forward, and the field is now moving faster than ever before.
Inspiration from nature
Andrew Mearns Spragg did a PhD in marine biotechnology and is the founder and CSO of Jellagen, a Welsh biotech company that is taking inspiration from the ocean and developing new bioactive compounds.
“I became increasingly aware of collagen and I was looking at a new way into accessing that market and also finding a new way into that technology,” Mearns Spragg explained.
“Through my marine networks, and through my entrepreneurial background, I was acutely aware that jellyfish seemed like a great opportunity to explore and offered something technologically new and unique, potentially because of their evolutionary ancient lineage.”
Jellagen was founded in 2015 and recently raised a £8.7 million ($10.6 million) Series A fundraising round. The company’s lead product is jellyfish Collagen Type 0, which is suitable for a variety of different uses in tissue engineering and regeneration.
Collagen is an important structural protein that helps maintain tissue structure and integrity. It is commonly used in medicine for cosmetic procedures and for applications such as wound healing. Currently, the majority of collagen is sourced from mammals such as pigs, cows and rats, which can carry disease if transferred to humans.
The jellyfish collagen does not cause this problem and appears to encourage more natural tissue healing with less inflammation.
“Through our published work, we now understand that our Collagen Type 0 offers good biocompatibility and tissue engineering potential validated in a number of in vivo models,” said Mearns Spragg. “For example, compared to traditional mammalian-derived collagens currently used for medical applications, we know that our jellyfish-derived collagen Type 0 promotes a much better tissue reaction, offering the potential of a more natural healing process.”
The company continues to develop its collagen products and has recently started a collaborative project to create a bio-ink with their collagen with another startup in Wales, Copner Biotech, which is designing technology to support 3D bioprinting for tissue engineering applications. It is not yet in clinical trials with its products, but has gathered a lot of preclinical data showing good efficacy.
“We’re now going through the process of proving to the regulators that our collagen meets the requirements of biocompatibility and safety to enable future product medical device registration,” explained Mearns Spragg.
AMSilk is another company pioneering natural biomaterials, in this case spider silk. The company engineers the DNA of bacteria to make them produce spider silk protein. Founded in 2008, the company is based near Munich in Germany and has already pioneered many products based on its spider silk including a biodegradable running shoe and lightweight materials for plane construction.
The spider silk developed by AMSilk is also a useful material for medical use, particularly for tissue regeneration and other medical purposes. “4,000 years ago there were the first stories about how to apply and use spider silk to close wounds or to support the wound healing process,” explained Tilmann Petersen, head of business development medtech at AMSilk.
“Spider silk proteins have different properties. Spiders can make many different fibers and use different kinds of compositions to achieve different kinds of benefits. We’re taking some of the properties and using it for very special cases.”
These applications include coatings for medical implants such as those used in breast implants. A very thin layer of silk protein on the outside of the implant can help increase the success of the implant by reducing chances for infection, as microbe growth is blocked by the physical barrier, or excessive inflammation.
“The bacteria and fungi cannot grow on it,” noted Petersen. “On the other hand, we have the wetting effect that its hydrophilicity is increased, so it also reduces the invasive stress for implantation.”
Image/Shutterstock
Other medical products the company is working on include gels that can be used as a wound dressing or for other purposes such as dermal fillers.
The French biotech Tissium (formerly Gecko Biomedical) is another company with a biomaterial product inspired by nature. Maria Pereira is a co-founder of the company and is currently its chief innovation officer. “When I started my PhD, the challenge that we got from a surgeon was that he wanted to repair holes inside the hearts of children through a minimally invasive approach,” she said.
“The challenge is that if you think about a beating heart, there’s a lot of fluid, there’s movement, it’s a very dynamic, complex environment and we had no materials available that could do what we wanted to achieve. What we set ourselves to do was to develop an adhesive that could be delivered minimally invasively and that could be used to repair these defects.”
Founded in 2013, Tissium has developed a surgical sealant inspired by the sticky secretions from slugs, snails and sandcastle worms. The key property of these secretions is that they are able to stick to surfaces, even when they are in a wet environment, something very useful during surgery for sealing wounds inside the body.
Tissium received a CE Mark for its vascular sealant in 2017 and has now developed a range of other products including glues for use in peripheral nerve and gastrointestinal procedures, 3D printed surgical scaffolds for nerve repair and adhesives that can deliver drugs to a specific tissue. It raised €50 million ($53.8 million) in Series C financing last year to fund the commercialization of new and existing products.
How to mend a broken heart
Naturally inspired and artificial biomaterials can be used to solve a variety of medical problems that are hard to tackle by other means. For example, several biotech companies and researchers are working to solve difficult-to-treat cardiovascular problems by combining tissue engineering with innovative biomaterials.
One such company is Xeltis, a company headquartered in Eindhoven in the Netherlands formed from the merger of a Dutch and a Swiss startup in 2006. The company uses artificial biomaterials, bioabsorbable polymers, to make artificial heart valves and blood vessels. They work by triggering the body to start repairing itself, but rather than healing a wound, the cells colonize and gradually replace the implants in a process called endogenous tissue restoration.
“We implant our devices and cells are able to permeate to nest inside the material. Then, the cells start making new tissue that fills the pores in between our implants. The cells also take care of the gradual resorption of our material,” explained Xeltis CTO and co-founder Martijn Cox. “You start with a medical device, a plastic cube, if you will, but what you end up with is the patient’s own natural tissue.”
The company’s lead device is a restorative pulmonary heart valve for right ventricular outflow tract reconstruction in children and young people born with heart defects, which is already being tested in a clinical trial. The big advantage of this type of approach is that the polymer valve is slowly replaced by cells from the patient over time and so the replacement valve can then grow with the children.
“What’s unique about our materials is that the supramolecular nature of them allows us to tune the speed at which the material degrades on the one hand, and we can also tune how strong or how flexible the material is,” said Cox.
“What’s key in our applications is that you have a balance between the strength or functionality of your implants versus the strength or functionality of the new tissue that’s forming. If the implant goes down in its strength, then the tissue goes up in its strength and this needs to be matched. You don’t want your implant to lose strength too fast. It needs to give the body time to replace it.”
Cox explained that before deciding on a bioabsorbable polymer implant approach, he and his colleagues had planned to grow heart valves in the lab and then implant the ready valve into the patient. However, they discovered this was very complex from both a technological and a regulatory point of view, as well as being prohibitively expensive.
“We learned that the materials that we were working with actually had the right properties to be able to skip the laboratory. We could implant the material directly into the body and then use the body as the bioreactor,” he explained.
The company is also applying its technology to producing aortic valve replacements and blood vessels for coronary artery bypass grafts and hemodialyisis vascular access grafts.
Nino Chirico, a PhD student in the lab of Professor Sluijter and Professor van Mil at the University Medical Centre in Utrecht, the Netherlands, is also working on mending damaged hearts. He and his colleagues have used advanced 3D bioprinting techniques to create a specialized patch comprised of a mesh made of the biodegradable polymer polycaprolactone, with cardiac cells encased inside, that can be placed on the outside of the heart to repair damage caused during a heart attack.
“Cells do not really like hard materials. They prefer soft materials and that’s why we created this blend where we actually place the cells inside the outer gel, but then the outer gel is kind of encapsulated inside this new mesh,” said Chirico. “This is able to provide mechanical properties which are similar to the human heart.”
Researchers in Germany are also working on a similar cardiac patch, but Chirico thinks that the specialized mesh structure of his group patch will help to encourage the cardiac cells to grow in the correct orientation, something that could help regenerate tissue structure in the heart and produce a better end result.
The Utrecht project is still at an early stage, but has the potential to help many people if shown to be effective and economically viable. “We are at the beginning… The idea is to move further with in vivo studies on pigs, and then hopefully to the clinic,” said Chirico.
Image/Shutterstock
Solving a sticky problem
Closing wounds in a quick and effective way with minimal tissue damage is something medical professionals have been striving to do for many years. While there are effective and long-used measures such as suturing or cauterization and, more recently, medical super glues, these methods are not perfect and so the search for better methods has continued.
A number of companies and researchers are applying novel biomaterials to this problem. For example, Tissium’s surgical sealant inspired by the secretions from snails and sandcastle worms.
“We needed something very hydrophobic, so it stays where the surgeon wants the material to be,” said Pereira. “If you look to snails, they produce these types of hydrophobic secretions that stay on a certain location and after that can be activated by certain stimuli, sometimes pH, that make it go from a liquid state to solid state and adhere to the underlying substrate… We had nothing available, so we looked to nature to try to understand what are the key properties we need to be able to achieve what we want to achieve.”
Matthew Harrington and colleagues at McGill are working on a different type of medical adhesive. Their product also has hydrophobic properties, but is inspired by mussels and the fibers they use to attach to rocks.
“The proteins are stored as a liquid crystal in these tiny little vesicles. Inside the membrane of these vesicles, you have a liquid crystal form of collagen… when the muscle wants to make a new fiber, which is essentially like a tendon, it just secretes them into a small channel,” said Harrington. “It goes from slightly acidic to basic, and makes them go from a liquid to a solid. In just a few minutes, you have this fiber, which is tougher than Kevlar.”
Harrington and colleagues also discovered recently that berries from the mistletoe plant also have promising adhesive properties and are assessing if this biomaterial could be developed for medical use.
Kibret Mequanint is a professor at the University of Western Ontario in Canada with a focus on biomaterials and tissue engineering. He has helped to develop a different type of wound sealant for use in emergencies involving a chemically modified gelatin and a type of snake venom, which is a known clotting agent. The gel-like material can be activated by visible light.
“Anyone these days is very likely to have a smartphone, you just basically turn the camera on, just on the wound,” explained Mequanint. “The light activates the gel, the gel sticks to the tissue and then the snake venom acts as a coagulant for the blood and so the bleeding stops.”
Future directions for biomaterials and tissue engineering
It is clear that many innovative biomaterials are being developed and tested for use in tissue engineering and regeneration, but what needs to happen before they can be used more widely in the clinic?
“In the biomaterials space there’s a lot of research, but it’s always been challenging to translate new biomaterials from the bench to the bedside,” said Pereira.
“What I would like to see is these discoveries reaching a point where they can benefit the broad population that requires them, but we are not there yet,” added Mequanint.
A few factors need to be addressed before biomaterials can be used more widely for tissue regeneration and engineering. One is cost. Many people are moving away from more complex and costly cellular therapies to devices such as those produced by Xeltis.
“You see more and more that people are moving away from cell-based approaches, just because it’s complex and expensive,” said Cox.
“Accessing venture capital (VC) funds can be a challenge as the focus of many VC firms may not be in the field of biomaterials,”explained Mearns Spragg. “That then limits how quickly young companies can access the market because it can cost significant sums of money to get materials to market.”
Other possible stumbling blocks are the complexity, which can require large multidisciplinary teams with a range of different specialties, as well as navigating potential regulatory pitfalls.
Tissue engineering is definitely being boosted by the creation of organoids and lab-on-a-chip devices, which are showcasing the power of the technology and helping to test new drugs and other therapies.
Demand for biomaterials has also increased, particularly for natural, sustainable biomaterials that can be used for medical applications such as AMSilk’s silk protein. “Device and implant manufacturers need to get rid of more and more non-sustainable products and replace them with sustainable products. [They] see our proteins as sustainable, as they degrade naturally without any harmful byproducts,” said Petersen.
Many products and therapies in the biomaterials and tissue engineering and regeneration space are at an early stage of development, but the field does seem to be speeding up and maturing.
“People are understanding biomaterials better and better, and understanding how to use these to harness the body’s innate capability of healing,” concluded Cox.
“The technological advances that have occurred over the last 20 years have definitely made it possible to make faster discoveries, and also to gain even deeper insights into connections of structure, chemistry and function,” added Harrington.